Theoretical Problems with Greenhouse-Gas Theory
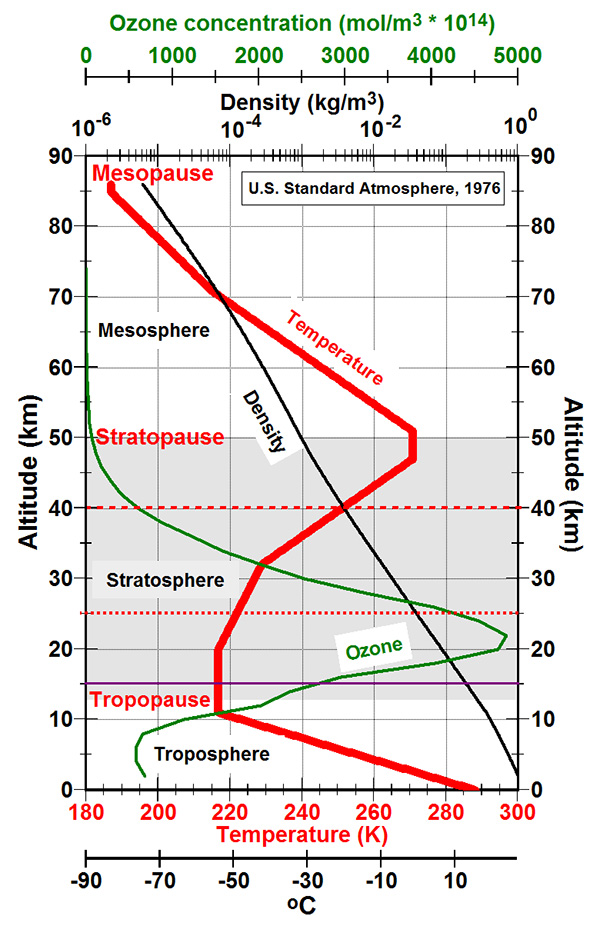
Mean surface temperatures on Earth result from a dynamic equilibrium resulting from Earth’s radiation balance between mean thermal energy received at the top of the atmosphere from the Sun and mean thermal energy radiated back into space from the Earth-atmosphere system. Simple calculations (Jacob, 1999, Section 7.2.2) assuming an observed mean albedo of 0.28, meaning that 28% of solar radiation is reflected back into space by clouds and matter on Earth, suggest mean surface temperatures should be around -18oC (-0.4oF). But observed temperature means are closer to 15oC (59oF). It has been assumed by most scientists that this 33oC temperature difference is caused primarily by greenhouse gases absorbing infrared thermal radiation emitted by Earth, heating the atmosphere, which then radiates back part of this heat to Earth, warming Earth.
This popular assumption ignores the well-observed reality that the atmosphere above the tropopause is heated primarily by solar ultraviolet radiation that causes photoionization and photodissociation as explained in The Primary Cause of WarmingThe Primary Cause of Warming. While the tropopause may be 70oC colder than Earth’s surface, the stratopause, roughly 35 km higher, is often 55oC warmer than the tropopause. This is the greatest warming observed anywhere in the Earth-atmosphere system. The atmosphere above the tropopause thus forms, in effect, a thermal blanket around Earth. The Second Law of Thermodynamics says that heat always flows spontaneously from hot to cold. Thus thermal energy flows away from Earth’s surface to the base of this blanket primarily via radiation, convection, and evaporation forming a linear temperature gradient described as the lapse rate, which averages a decrease in temperature of around 6.4oC per kilometer of altitude. Thus Earth loses heat through the troposphere at a rate that results in this lapse rate.
The lapse rate is observed to approach 10oC/km for a very dry troposphere and 5.5oC/km for a very wet troposphere. Thus the troposphere is warmer in the presence of water, the primary greenhouse gas. But water improves the efficiency of heat transfer away from Earth through evaporation, condensation, and convection. Whether greenhouse gases such as carbon dioxide (CO2) and methane (CH4) affect the lapse rate substantially has not actually been demonstrated. Since air in polar regions is much drier than air in tropical regions, moisture content may have a lot to do with the height of the tropopause ranging from 9 km at the poles to 17 km at the equator.
The sensitivity of climate to greenhouse gases has traditionally been calculated by assuming most observed changes in mean temperature are caused by changes in the concentrations of greenhouse gases, e.g. PALEOSENS Project Members (2012), Gillett et al. (2012), Schmittner et al. (2011), and Frank et al. (2010). One should be able to put CO2 gas in a cylinder, pass infrared radiation through the gas, and measure the amount of energy absorbed and any increase in temperature for different concentrations. It should also be possible to measure whether absorption saturates. I have been unable to find any such experiments documented in the literature and have even asked some of the best historians of greenhouse gas theory if they knew of any such experiments. John Tyndall (1861) and others have demonstrated that carbon dioxide absorbs radiant energy. Knut Ångström (1900) concludes: “Under no circumstances should carbon dioxide absorb more than 16 percent of terrestrial radiation, and the size of this absorption varies quantitatively very little, as long as there is not less than 20 percent of the existing value.” Modern spectral physicists have measured with great precision the spectral lines of absorption (Rothman et al., 2013), but no one is documented as having measured the change in temperature resulting from such absorption.
The Intergovernmental Panel on Climate Change (IPCC) defines the greenhouse effect in the Glossary of the Climate Change 2007: Synthesis Report as follows:
Greenhouse gases effectively absorb thermal infrared radiation, emitted by the Earth’s surface, by the atmosphere itself due to the same gases, and by clouds. Atmospheric radiation is emitted to all sides, including downward to the Earth’s surface. Thus greenhouse gases trap heat within the surface-troposphere system. This is called the greenhouse effect. Thermal infrared radiation in the troposphere is strongly coupled to the temperature of the atmosphere at the altitude at which it is emitted. In the troposphere, the temperature generally decreases with height. Effectively, infrared radiation emitted to space originates from an altitude with a temperature of, on average, –19°C, in balance with the net incoming solar radiation, whereas the Earth’s surface is kept at a much higher temperature of, on average, +14°C. An increase in the concentration of greenhouse gases leads to an increased infrared opacity of the atmosphere, and therefore to an effective radiation into space from a higher altitude at a lower temperature. This causes a radiative forcing that leads to an enhancement of the greenhouse effect, the so-called enhanced greenhouse effect.
This and similar definitions as well as the computer codes used to calculate radiative forcing and to model climate are based on many assumptions including:
- Enough energy is involved in greenhouse gas absorption to cause the observed global warming
- Absorption of infrared radiation by carbon dioxide is not saturated
- Air behaves similar to a black body of matter radiating energy in all directions
- Energy absorbed by CO2 primarily raises the temperature of air
- Radiation from a colder body of air warms a warmer Earth
- Oceans absorb infrared radiation from the atmosphere relatively efficiently
- The effective power of radiation in watts per square meter is calculated by integrating across a broad band of wavelengths
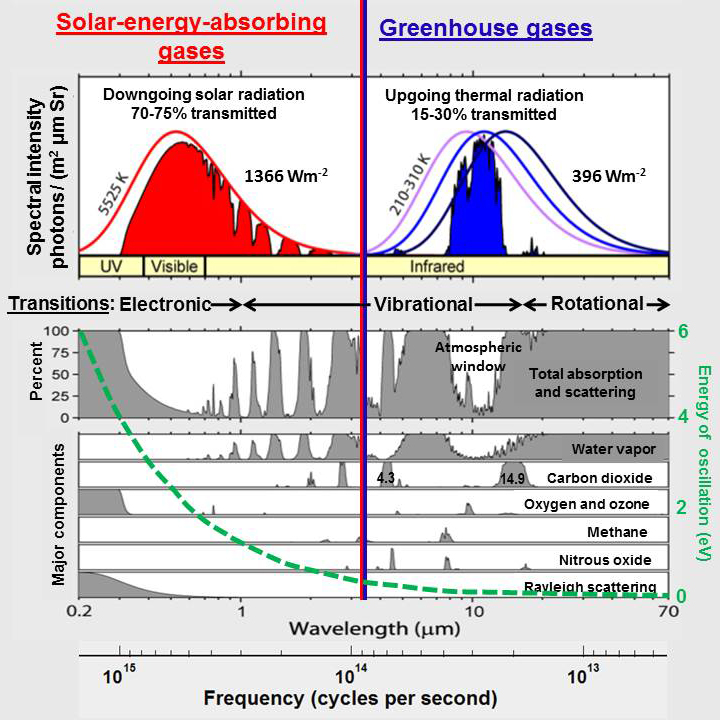
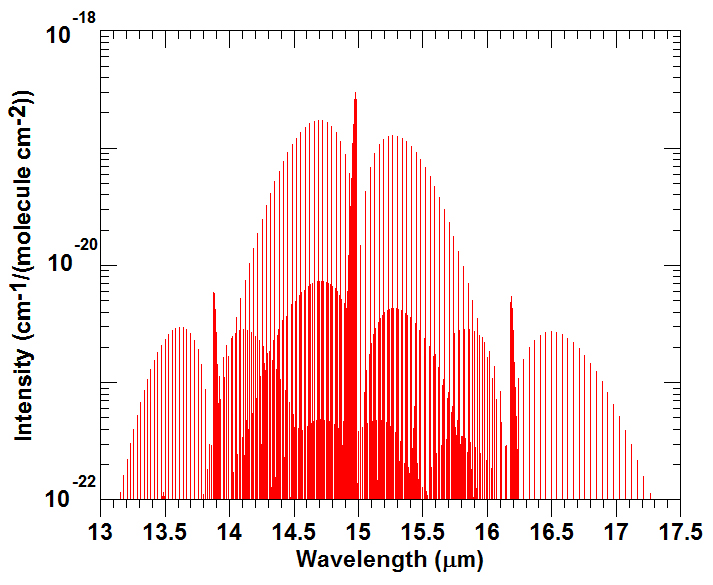
(1) The blue line to the left shows the spectral radiance, the amount of radiation at each wavelength, for infrared energy radiated by Earth at a temperature of 260K according to Planck’s law. The red line shows the spectral radiance for solar radiation at the top of Earth’s atmosphere. The solar and terrestrial curves have been normalized to have the same height. Peak spectral radiance of solar radiation at the top of the atmosphere is actually 69 times larger than the peak spectral radiance for terrestrial radiation. The blue shaded area shows the spectral radiance for terrestrial radiation reaching space while the red shaded area shows the spectral radiance for solar radiation reaching Earth.
The gray shaded areas show the percentage of absorption and Rayleigh scattering that occur at each wavelength. Note that water vapor absorbs energy over a wide variety of wavelengths while carbon dioxide only absorbs energy in a few wave bands. The band around 4.3 micrometers (μm) does not overlap with a water vapor absorption band but contains little infrared energy. The band around 14.9 μm overlaps absorption by water vapor substantially, although the spectral lines (Rothman et al., 2013) for the two gases do not overlap much in detail. Concentrations of water vapor range from 0.1 to 5% while concentrations of CO2 are less than 0.04%. Absorption by all greenhouse gases is along very narrow spectral lines shown in the figure to the right for the CO2 peak near 14.9 μm (Rothman et al., 2013). Thus CO2 absorbs only a fraction of the energy within its narrow bands of absorption. CO2 absorbs only a very small amount of the broad spectrum of infrared heat (energy) radiated by Earth.
(2) Energy is transferred from the electromagnetic field of radiation to the molecule when oscillations within the field cause a particular degree of freedom of the bonds among the atoms to resonate, increasing the internal energy of the molecule. If the degree of freedom is oscillating at the same intensity (spectral amount) as the field, the molecule is saturated, meaning that it cannot absorb more of that particular energy until it loses the energy already absorbed. Loss is assumed to occur during the numerous collisions of gas molecules in the atmosphere but the details of that loss are not well documented.
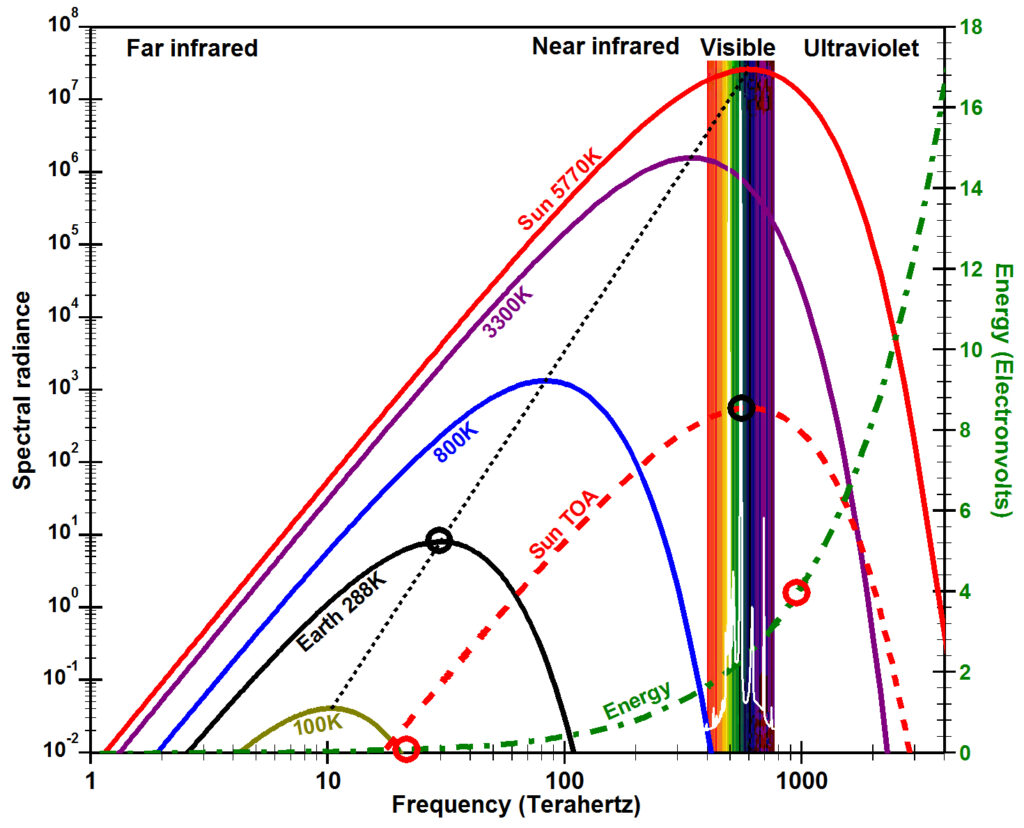
(3) Electromagnetic radiation, the spectral radiance, emitted by a black body of matter contains a broad spectrum of frequencies (wavelengths) dependent on the temperature of the surface of the body as described by Planck’s law (figure left). A black body is a body of matter that absorbs all incident radiation regardless of frequency or angle of incidence. Whatever the frequency spectrum of incident radiation, temperature for a black body is not defined until internal oscillations share their energy through conduction to reach thermal equilibrium. Then the frequency spectrum of radiation from the surface of the black body approaches the shape of a Planck curve. Temperature in matter is a macroscopic property of microscopic oscillations that share energy through conduction.
Temperature of a gas, on the other hand, is observed to be proportional to the mean translational velocity of the molecules and atoms making up the gas. The molecules cannot share their energy via thermal conduction except by the reality that a gradient in temperature must be accompanied by a gradient in the translational velocities of the gas molecules. A layer of gas in the atmosphere cannot be considered to be a black body because only some of its constituents absorb only very narrow frequency bands of incident energy dependent on the physical properties of primarily its triatomic constituents and because there is no radiant surface of the gas. Individual gas molecules can emit radiation through fluorescence, but that only occurs when electronic transitions are involved where an electron moves from a higher to a lower energy state. Infrared radiation does not contain enough energy to cause electronic transitions. It has yet to be shown that a layer of air in the atmosphere emits radiation described by Kirchoff’s law of thermal radiation or Stefan’s law. Downgoing longwave, infrared radiation is observed at Earth’s surface, but the effect of changing concentrations of CO2 on this radiation has not been demonstrated.
Fourier (1822, p. 28) explains radiation from the surface of a body as follows: “Suppose that a body bounded by a plane surface having a certain area (a square meter) is maintained in any manner whatever at constant temperature 1, common to all its points, and that the surface in question is in contact with air maintained at temperature 0: the heat which escapes continuously at the surface and passes into the surrounding medium will be replaced always by the heat which proceeds from the constant cause to whose action the body is exposed; thus a certain quantity of heat denoted by h will flow through the surface in a definite time (a minute).” If the surface of a molecule of gas loses heat, there is no way by conduction for this heat to be replenished from other molecules of gas. Thus if there was some way for molecules of gas to radiate infrared energy, the radiation would not last long enough to be detected. A layer of gas does not appear to radiate energy as if it were a black body of matter.
(4) Is is assumed that the radiant energy absorbed by CO2 and other greenhouse-gas molecules is mostly converted to temperature of the gas through numerous collisions. But the oscillatory energy in the radiation field increases the internal energy within the molecule. The molecules can only share energy through collisions exchanging primarily translational velocity. It is not clear how much internal energy of oscillation within molecules is shared in such collisions nand thus how much the temperature of air is raised.
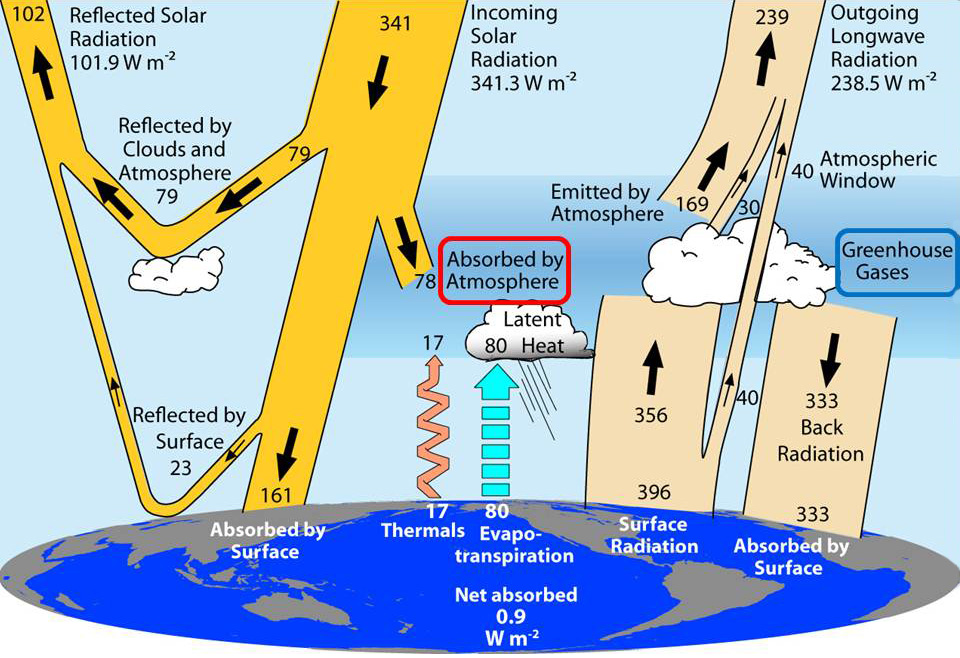
(5) Trenberth et al. (2009) and Trenberth and Fasullo (2012) estimate gloabal energy balance at shown in the figure to the right. They calculate that 84% of terrestrial radiation is radiated back from the atmosphere to Earth or 64% of all energy absorbed by the atmosphere is radiated back to Earth. (Wild et al., 2013) show similar results. If radiation by the atmosphere is in all directions, then only 50% of the energy absorbed could possibly be radiated downward. But a more serious problem is the assumption that radiation from a colder atmosphere is absorbed by a warmer Earth, increasing the temperature of the warmer Earth. The Second Law of Thermodynamics explains the observation that heat always flows spontaneously from regions with higher temperature to regions with lower temperature, never in reverse. This is true in matter and for radiation. You do not stand next to a cold stove in order to warm up.
Note from Planck’s law that the spectral radiance (figure above left) emitted by a warmer black body is higher at all wavelengths and much higher at shorter wavelengths than the spectral radiance emitted by a cooler black body at the same distance. Thus the spectral radiance from a cooler black body does not contain sufficient energy to increase the temperature of a warmer black body. This gets down to the specific mechanism by which radiation is absorbed. Radiation is simply oscillatory energy absorbed most likely through resonance. If the amplitude of internal oscillations at a specific wavelength (frequency) is less in the radiation than in the black body, energy (heat) cannot flow spontaneously from the radiation to the body. It would be reflected. Much of the cosmic microwave background could simply be the energy without sufficient intensity or brightness to be absorbed by available matter.
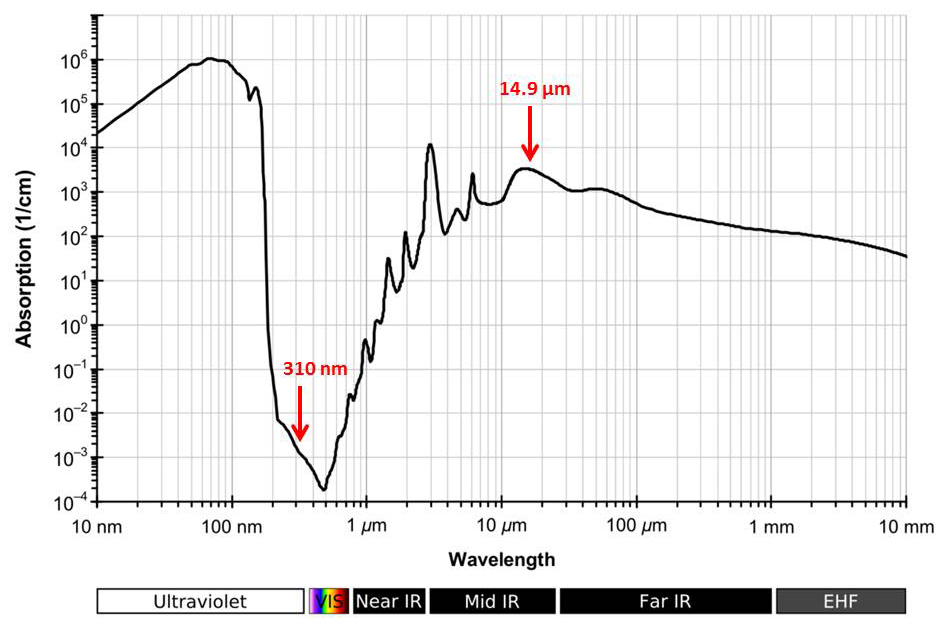
(6) Infrared radiation in the 14.9 μm range that is absorbed strongly by CO2 is absorbed by liquid water more than 6 orders of magnitude more strongly than ultraviolet radiation around 310 nm that is able to penetrate the atmosphere when ozone is depleted (red arrows, figure left). Infrared radiation barely penetrates the surface of the ocean (Clark, 2010) (Morel, 1974, pdf). While it may get mixed in just below the surface, it is quite likely to be radiated back into the atmosphere at night. The efficiency of infrared energy in warming the ocean is very low compared to efficiency of ultraviolet radiation that penetrates at least 10 meters (Tedetti and Sempéré, 2006) into the oceanic mixed layer.
(7) Planck (1900), in order to write his law, had to postulate that the energy (E) in a field of radiation is equal to Planck’s constant (h) times the frequency of the radiation (ν, the Greek letter nu) (E=hν). But this is only for one frequency; what about the broad range of frequencies (wavelengths) available in solar and terrestrial radiation and involved in the definition of temperature shown by Planck’s law (figure left above)? They matter little, because global warming is not about an integral of all the energy available, it is only about the available energy that is utilized. The Planck postulate (E=hν) can be thought of as the energy contained within radiation that causes chemical change, such as photodissociation of a molecule. This very simple equation led to the concept that energy is quantized, which led to Einstein’s light quantum (1905), Lewis’s photon (1926), and quantum mechanics in general. But this simple equation is still not well understood until we realize that it is the physical properties of the molecule that determine what available energy is utilized. A molecule of oxygen may absorb many different frequencies of radiant energy, but it is only those frequencies greater than 1237 Terahertz (wavelength <242.4 nm, energy >5.1 eV) that cause photodissociation and warming of the atmosphere. Other energy may or may not be absorbed, but its effect on temperature is much less than photodissociation. When stratospheric ozone is depleted, more of the energy that photodissociates ozone can reach Earth and be absorbed by the ocean. Through conduction, this energy ends up warming the ocean.
Energy extracted from the field of terrestrial infrared radiation by a molecule of carbon dioxide, is 69 times less than the thermal energy released by photodissociation of a molecule of ozone (red circles along the dashed green curve showing energy in the second figure above left). There simply is not enough energy involved in greenhouse gases, even if it all were converted to heat and even if it all were absorbed by Earth, to warm Earth.
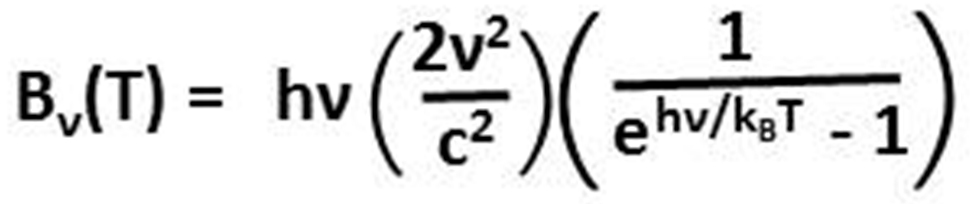
The fundamental problem with greenhouse-gas theory
Atmospheric scientists currently quantify the effects of greenhouse gases on global warming, the radiative forcing, by integrating spectral radiance across all frequencies under a Planck curve assuming, in effect, that temperature is increased primarily by the amount of radiation, rather than by increased frequency contained within radiation. Planck’s law (right) can be expressed as energy hν times two terms whose product is the amount of energy at that frequency. The problem is that for radiation, the first term is the color of light (the energy) which is well observed not to change over galactic distances except for Doppler effects while the next two terms represent the amount of light that is well observed to decrease as a function of one over the distance squared as the energy radiates away from the source. Temperature in matter is primarily a function of the frequency (energy) of oscillation received via radiation (first term in the equation), not the amount (second and third terms).
The family of Planck curves for spectral radiance plotted above left show that to increase the temperature of a body, you must not only increase the amount of oscillation at each frequency but you must also increase the frequency of the maximum amount of spectral radiance, the dashed black line representing Wien’s law. A huge amount of radiation from a colder black body simply does not have the frequency content to raise the temperature of a warmer black body. The radiation from the colder black body is most likely not even absorbed by the warmer black body because heat flows via resonance from higher spectral amount to lower spectral amount at each frequency. But even if the radiation is absorbed, conduction cannot increase the frequency (energy) of the oscillations that are approaching thermal equilibrium. Conduction can spread the energy out among lower frequencies but it cannot increase the energy.
Energy radiated by Earth, no matter how it is absorbed and radiated from the atmosphere, cannot warm Earth. The maximum frequency of this radiation is the same as or less than the maximum frequency of oscillations at Earth’s surface and thus cannot warm Earth. Ultraviolet energy from the sun, on the other hand, received on Earth during times of ozone depletion, is oscillating at frequencies on the order of 1000 Terahertz, more than 30 times greater than the peak frequencies of radiation from Earth and thus warms Earth very effectively. Ozone depletion plays the dominant role in global warming.