What is Electromagnetic Radiation?
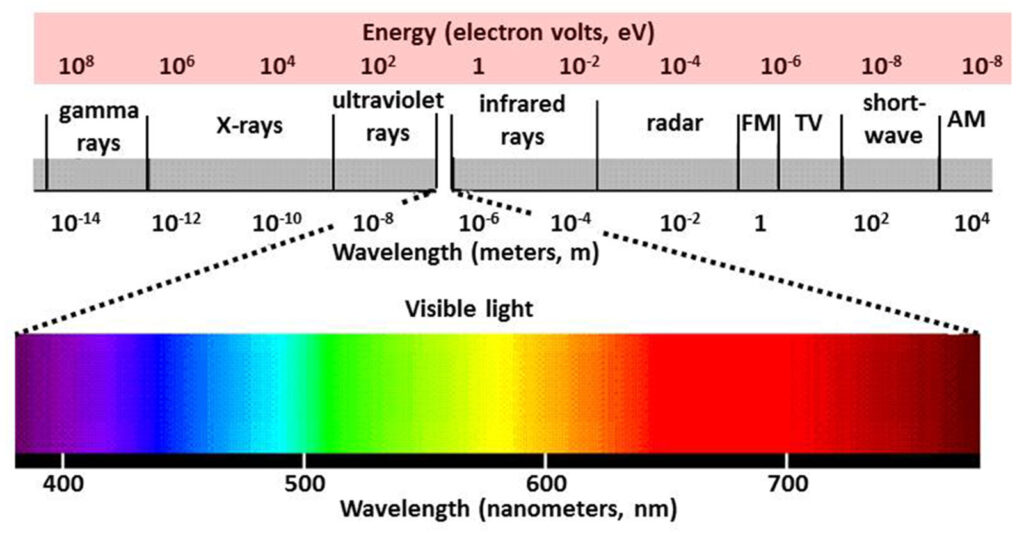
Climate is driven primarily by electromagnetic radiation (EMR) from the Sun. Climate change appears to occur primarily when more or less than normal amounts of solar energy are either absorbed or reflected by Earth’s atmosphere changing the amount of energy reaching Earth’s surface. What physically is EMR and how is it absorbed and reflected? Let’s use the word light as shorthand for the much broader spectrum of EMR because we all have good physical intuition about light. Brightness or amount is more intuitive than spectral radiance. The physics should be similar even though energy varies from less than 0.001 eV for the far-infrared to 2 or 3 eV for visible light, to more than 100 eV for the extreme ultraviolet. Visible and near ultraviolet light play the primary role in heating Earth.
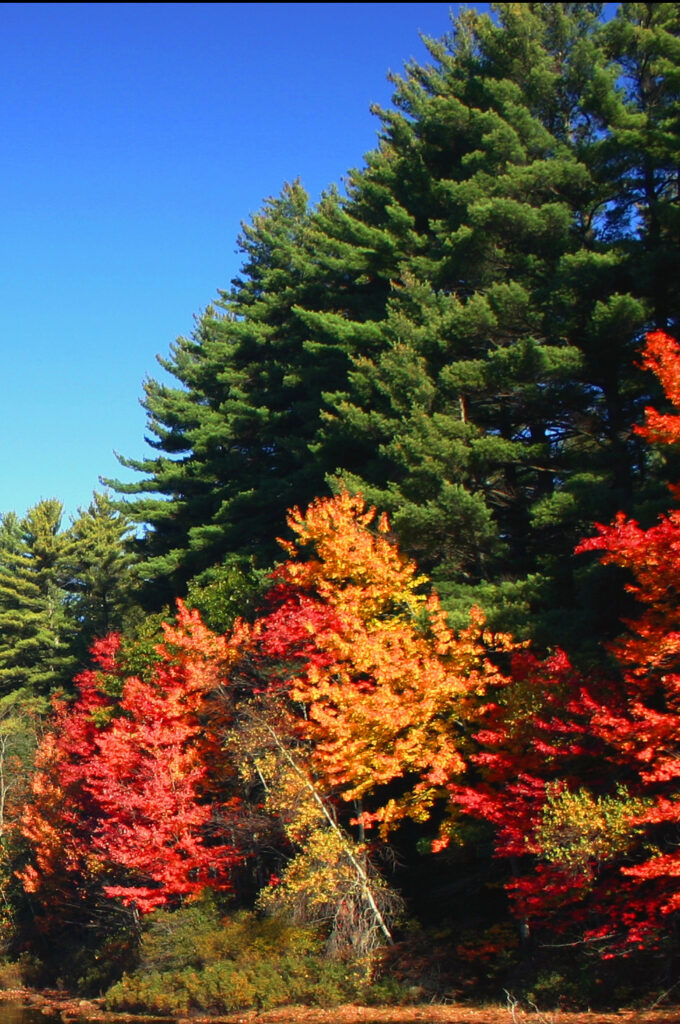
At any point in space, in a gas such as air, in a liquid, or in a translucent solid, light consists of a spectrum of frequencies (colors) where each frequency can be thought of as having spectral amount (brightness). This spectrum appears to travel the shortest distance between source and receiver, a straight line that we think of as a ray. When we look at colorful fall foliage, for example, each molecule or cell of everything we can see absorbs most radiation received from the Sun and is warmed much like a pot on a stove. But the molecules or cells responsible for color are caused to resonate, radiating a very narrow frequency spectrum, their color. This radiation has a brightness determined by the brightness of the light illuminating matter. The brightness goes to zero as the amount of incoming light goes to zero, causing the molecule to no longer radiate visible frequencies bright enough to see, although the molecule continues to radiate infrared frequencies based on the temperature of the matter that it is part of. The radiation from each molecule received by our eyes, photographic film, or the CMOS sensor of a digital camera, for example, causes a photochemical reaction and the amount of that reaction is defined by the spectral amount (brightness) received at that specific frequency. Our field of view is made up of so many of these “rays” that we can resolve the intricacies of color with very high resolution, yet these rays of light do not interfere with each other until they interact with matter. We take all this for granted, but it feels like “spooky interaction at a distance,” Einstein’s description of quantum entanglement. The energy of oscillation in one piece of matter influences the energy of oscillation in another piece of matter at an arbitrarily large distance separated by a gas such as air or by the vacuum of space. This simple “entanglement” is a physical property of an electromagnetic field. Quantum entanglement, even at speeds much greater than the velocity of light, has taken on far more complicated mathematical properties that are actively debated through the different interpretations prevalent in quantum mechanics.
Light illuminates matter, but light itself is not visible, it is dark, until it interacts with matter. Given that Earth receives less than 5 x 10-8 % of Sun’s radiation, there must be a lot of dark energy in space that changes in time only if the rate of conversion of mass to energy in stars and elsewhere changes locally as it interacts with matter, such as in the shadow behind a planet.
The widespread existence of dark energy suggests that the medium light travels in is light itself, i.e. there is an electromagnetic field located everywhere that permeates space, atmospheres, and matter in three dimensions. The physical properties of this field vary in time if the sources of energy vary. The spectral amount decreases proportional to one over the square of the distance from the source. Energy in the form of frequency of oscillation appears to radiate outward from matter, traveling through this field along what is thought of as a straight line from source to receiver, although light rays can be bent very slightly by gravity, currently thought of as distortions in spacetime (Einstein, 1905), and more strongly as they interact directly with matter. Energy is either extracted from this field by matter or the presence of matter changes the local physical properties of this energy field.
Planck’s postulate (E=hν) says that light is simply energy in the form of frequency (cycles per second) whose existence seems to depend on the continual interaction between an electric field and a magnetic field, suggesting that what we think of as the velocity of light must be related to the rate that an electric field can induce a magnetic field, which in turn can induce an electric field, ad infinitum. There is no known medium (luminiferous aether) in space and there are no physical observations that show that light in space is either a wave or a particle, that it has wavelength or mass, because there can be no observation until light interacts with matter.
Light can travel inter-galactic distances without any change in frequency (except Doppler effects) and thus without any loss of energy, even though light diverges so that the amount of light, the brightness, is inversely proportional to the square of the distance from the center of the source to the receiver. Thus for light in space, the energy contained in light and the amount of light (brightness) are separate variables commonly multiplied together in order to determine spectral radiance. This is distinctly different from the energy contained in waves within matter where the wave energy works against the bonds holding matter together so that energy at a given location is proportional to the square of the amplitude of the wave at that location. Light does not travel in space in exactly the same way that waves travel in matter, although light is observed to interact with matter in a wavelike manner. Thinking of light in space as having the physical property of wavelength may be very misleading.
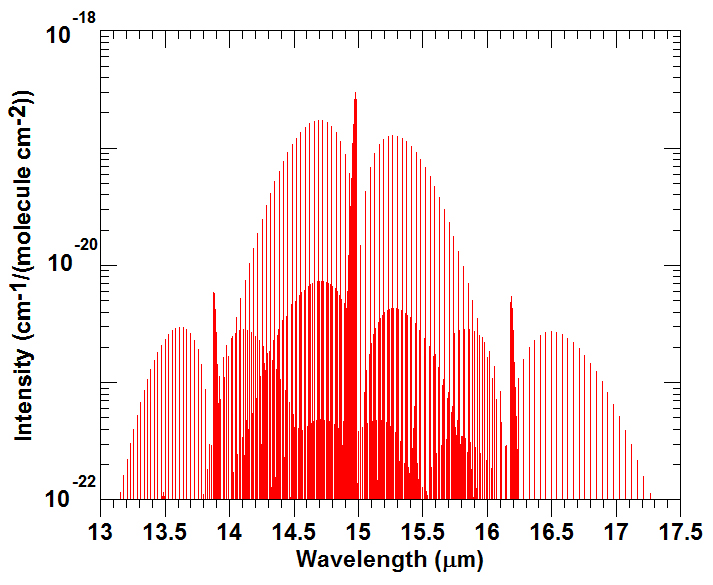
Thus light might be described most precisely as field-particle-wave triality or vacuum-gas-solid triality to emphasize that the physical properties of light are different in space, in atmospheres, and in matter. In the vacuum of space, light is an energy field extending in three dimensions, affecting the behavior of matter in the presence of the field. The frequencies of light do not change over galactic distances except when there is motion between source and receiver, but the spectral amount is inversely proportional to the distance between source and receiver squared. Light from one source does not interfere with light from a separate source except in the presence of matter.
Light travels similarly through a gas such as air except some of the molecules of gas absorb certain frequencies of light in a particle-like manner. Each degree of freedom of motion among and within atomic components of a gas molecule resonates at its natural harmonic frequencies, extracting spectral amount at those frequencies from the light field, adding the energy to the internal energy of the molecule. The energy absorbed is along very narrow spectral lines (Rothman et al., 2013), implying high-Q classical sympathetic resonance perhaps similar to that observed when undamped strings of a piano resonate to specific frequencies of sound in the room. The spectral components of the energy absorbed are determined by the physical properties of the molecule. Energy absorbed through rotational and vibrational transitions is shared among surrounding atoms/molecules via collisions. Energy absorbed through electronic transitions typically causes what we think of as an electron to resonate at a higher frequency (energy) similar to overtones on a vibrating string and this energy can be re-radiated typically through fluorescence when the atom/molecule returns to ground state often initiated by a collision. When these higher energy states are energetic enough, they can cause dissociation and even ionization, destroying the molecule.
The net energy transferred from the field to the gas molecule can be viewed as spectral amount for each frequency times Planck’s E=hν (Planck, 1901) integrated across all frequencies, or, for simplicity, Einstein’s light quantum (Einstein, 1905), or Lewis’s photon (Lewis, 1926). But the detailed nature of this spectrum of energy is determined by the physical characteristics of the receiver, not the physical characteristics of the source. Therefore, it does not make physical sense to talk of photons propagating from the source. The photon is a very useful mathematical concept central to quantum mechanics that is shorthand for a complex distribution of frequencies (energies and amounts) transferred from an electromagnetic field to matter.
Atoms and molecules constituting a gas can be thought of as similar to radio antennas tuned to very specific frequencies. Each has a different orientation so that each responds slightly differently to the surrounding electromagnetic field. If we knew all the details about the state of every molecule and atom and the state of the field, we could determine which molecules will interact when and how with the field. But this is impractical given the very large number of atoms/molecules involved leading to the statistical nature of quantum mechanics.
When light interacts with matter, defined as an assemblage of atoms and molecules bound by attractive forces, it can be reflected, refracted, diffracted, polarized, dispersed, focused, and/or attenuated because the energy in light works against the bonds holding matter together. The oscillations of light when interacting with matter cause waves in matter whose properties are determined by the nature of the matter such as the index of refraction, thought of as the ratio of the velocity of light in space to the velocity of light in matter. Light is attenuated in matter showing that energy and amount are now intermixed. Light in space and typically in air can be split by matter in a double-slit experiment (Young, 1802) where the width and spacing of the slits are small, causing the two rays to interfere, but rays from different sources of light do not interact until they both encounter the same piece of matter.
Sound, on the other hand, travels in air as compressional waves with a spectrum of frequencies emitted by oscillations at the source that cause the hair cells in the cochlea of the inner ear to resonate. The frequencies of sound do not change with distance and direction, except for Doppler effects, but spectral amounts from different sources are combined within air and are attenuated as the wave front spreads and as energy is absorbed by air, especially in the high frequencies.
Energy fields may be as large as the universe or as small as an electron or the fields that hold parts of atoms together. The diameter of a hydrogen atom is 145,000 times larger than the diameter of its nucleus. The vast majority of any atom is an energy field that we describe in terms of electrons and that de Broglie (1929) suggested consists of “stationary” waves in order to include “the concept of periodicity” (frequency). One could argue that everything consists of combinations of fields and the tiny components of matter, and that time (change) is made possible by the conversion of energy to matter and the conversion of matter to energy.
Natural scientists have debated since the time of Ancient Greece whether the energy contained in electromagnetic radiation (light) travels as waves or particles. By 1900, most physicists thought that radiation traveled as waves according to Maxwell’s equations (Maxwell, 1873) as demonstrated by Young’s famous double-slit experiment (Young, 1802), but they could not decide what type of matter these waves traveled in. Planck’s quantization of energy (E=hν) and Einstein’s light quantum led to the development of quantum electrodynamics (QED) based on the wave-particle duality of electromagnetic radiation emitted by an ensemble of harmonic oscillators. QED is arguably the most successful mathematical theory in physics in terms of explaining and predicting observations, but it is non-deterministic and leads to many concepts that do not make physical sense, something that plagued Planck and Einstein for the rest of their lives.
Light in space does not have the physical properties of wavelength or mass. Recognizing that electromagnetic radiation is simply a spectrum of frequencies (energies) that induces particle-like behavior in atoms/molecules of gas and wave-like behavior in matter is likely to make QED more deterministic, give us more physical insight into many of its conclusions, and bring us closer to understanding Nature.